US
Mid-Century Strategy for Deep Decarbonization
While CO2 accounts for four-fifths of U.S. greenhouse gas (GHG) emissions,
the remainder are highly potent heat-trapping gases, many of which have
near-term climate impacts due to their shorter “lifetimes” in the atmosphere.
Figure 6.1 shows the contribution of non-CO2 U.S. GHG emissions and their
major sources, including:
- methane (CH4) (55 percent),
- nitrous oxide (N2O) (31 percent),
- hydrofluorocarbons (HFCs) (13 percent), and
- other fluorinated gases such as PFCs, SF6, and NF3 (1 percent) (EPA
2016a).
Absent significant innovation and policy, non-CO2 GHG emissions are projected
to increase rapidly. For example, growing global demand
for food would drive broader use of nitrogen fertilizer and increased
livestock production, resulting in greater N2O and methane emissions.
A growing global population will also increase
demand for energy and refrigerants, leading to greater emissions of methane and
HFCs in the coming decades.
Additional challenges to mitigating non-CO2 GHG emissions include the diffuse
nature of their sources such as individual cattle
or air conditioners and the difficulty of detecting
and monitoring leaks.
Increased research, development, and demonstration (RD&D) is needed to
address these challenges.
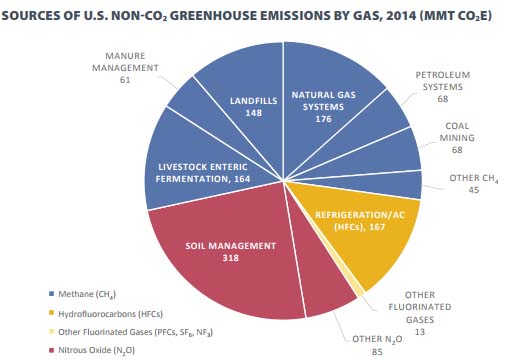 |
In order to compare the climate impacts of
each greenhouse gas, scientists use a “global warming potential” (GWP)
factor to convert the warming impacts from a non-CO2 gas into carbon dioxide
equivalent (CO2e). A GWP assumes a given time period, since different gases
have different lifetimes in the atmosphere.
Non-CO2 greenhouse gases are more potent than CO2 at trapping heat within
the atmosphere, and thus have high GWPs.
Using the IPCC AR5 GWP estimates for a 100-year time scale,
- methane is
28- 36 times more powerful than CO2,
- nitrous oxide
is 265-298 times more powerful, and
-
hydrofluorocarbons have GWPs as high as thousands or
tens of thousands (EPA 2016b).
Because methane and HFCs cycle out of the atmosphere more
quickly than CO2, their 10 and 20-year GWPs are even higher. This also means
that near-term global actions on non-CO2 GHG emissions can effectively
reduce the rate of near term warming. |
|
|
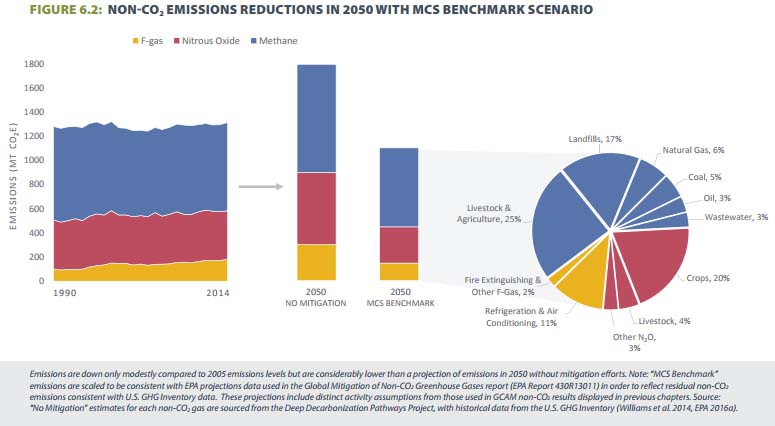
Emissions are down only modestly compared to 2005 emissions levels but are 50
percent lower than a projection of emissions in 2050 without mitigation efforts.
The MCS analysis of non-CO2 mitigation potential does not account
for major technological advances that may be achievable with increased RD&D
investment. The cost estimates used in the analysis assume only minor
technological progress over the next few decades. With continued innovation and
well-designed policies, we can achieve even deeper non-CO2 reductions than those
displayed in the MCS analysis.
As seen in Figure 6.2, the largest share of residual emissions in 2050 is
methane from livestock, landfills, and fossil fuel production. Other major
sources in 2050 include HFCs from existing equipment and appliances and N2O
emissions from crop production.
METHANE FROM FOSSIL FUEL SYSTEMS
Fossil fuels are not only the primary source of CO2 emissions but also a
major source of methane emissions. Methane is released across the supply chain
as part of fossil fuel production, processing, transmission, storage, and
distribution. These emissions are both intentional
(venting) and unintentional (leaks). Current estimates
attribute nearly one-third of total U.S. methane emissions to oil and natural
gas systems.
Coal mining also releases methane trapped in coal seams, accounting for
9 percent of U.S. methane emissions (EPA 2016a).
Decarbonizing the energy sector, including transitioning away from fossil fuels
to low-carbon energy, will not only reduce CO2 but will also reduce methane
emissions associated with fossil fuel extraction and processing. However, fossil
fuels will continue to play a role in the U.S. energy mix for some time. The MCS
therefore envisions additional measures to reduce methane from oil and gas,
including increasing the stringency of current standards and enhancing
investments to improve methane emissions measurement, capture, and repair
technology. Some of these methane reductions can be achieved highly cost
effectively with the recovery and sale of captured methane
The United States has already taken action to better identify and reduce methane
emissions from the energy sector. In 2014, President Obama released a national
methane strategy targeting the largest sources of methane, including from oil
and gas production and coal mines. The strategy also identifies opportunities to
reduce methane from agriculture and landfills, which are discussed in subsequent
sections. In 2015, the Obama Administration set a goal of reducing methane
emissions from the oil and gas sector 40 to 45 percent below 2012 levels by
2025. Canada and Mexico have since also committed to this goal. In recent years,
new information from studies of the U.S. oil and gas industry have indicated
that methane emissions are much higher than previously understood. EPA updated
the U.S. GHG Inventory with this information, resulting in a large increase in
its estimates. In May of 2016, EPA finalized the first-ever standards for
methane emissions from new and modified oil and gas facilities, and took the
first steps in the process of developing emissions standards for existing
sources.
Federal agencies are also coordinating a range of voluntary programs and
supporting industry efforts and research initiatives to reduce methane emissions
by recognizing leaders, through efforts like the Methane Challenge Program and
the Oil and Gas Methane Partnership of the Climate and Clean Air Coalition.
Additional federal programs are improving measurement and monitoring of oil and
gas sector emissions, such as ARPA-E’s MONITOR program, which has invested $30
million to help reduce the cost of detecting and quantifying natural gas leaks
(DOE 2014).
Recent evidence indicates that a small fraction of sources may be responsible
for a large portion of total oil and gas methane emissions (Brandt, Heath, and
Cooley 2016; Zavala-Araiza et al. 2015). Therefore, developing and deploying
monitoring capabilities to identify these sources may be particularly effective
for targeting mitigation actions. However, doing so is currently challenging due
to the fact that these high-emitting sources are dispersed across the United
States and may emit intermittently. Continuous monitoring at a large spatial
scale via remote sensing technologies could help identify these sources. Use of
new satellite, aircraft, and drone capabilities coupled with on-site continuous
monitoring and automated infrared imaging have the potential to greatly improve
leak detection, monitoring, and repairs.
In coal mining, commercially available technologies
can recover and reduce methane emissions. These mitigation technologies include
drainage and recovery systems to remove methane from the coal seam before mining
or from the area post-mining, destruction of ventilation air-methane, and
end-use application for recovered gas (e.g., electricity generation or use as a
process fuel for on-site heating).
METHANE AND NITROUS OXIDE FROM AGRICULTURE
Agricultural production contributes over 40 percent of U.S. non-CO2
greenhouse gas emissions in the form of N2O and methane. Agriculture, in
particular the use of nitrogen-rich fertilizers to increase crop yields, is the
source of three-fourths of annual U.S. N2O emissions. Agricultural methane
emissions are largely driven by livestock manure and enteric fermentation (EPA
2016a).
As discussed previously, global demand for food is projected to lead to greater
global agricultural-related methane and N2O emissions. In spite of this growth,
the MCS analysis points to potential actions to reduce N2O emissions
significantly from 2005 levels by 2050. Still, without additional technological
innovation, agricultural methane emissions will likely remain a significant GHG
source in 2050.
Many technologies and practices are currently available that can reduce methane
and N2O emissions associated with agricultural operations. Farmers, ranchers,
and land managers across the United States are already using many of these
techniques. Through the Department of Agriculture and programs such as the
Environmental Quality Incentives Program (EQIP), the United States has promoted
increased education, dissemination of online tools, and technical assistance to
help farmers manage livestock herds, improve manure management, modify animal
diets, and adopt alternative techniques to fertilizer applications. These
actions reduce emissions while also maintaining yields and decreasing costs. In
2015, the USDA announced its Building Blocks for Climate Smart Agriculture and
Forestry, previously discussed in Chapter 5. Through the Building Blocks, USDA
is working closely with farmers, ranchers, and rural communities to implement
voluntary, incentive-based practices that improve environmental conditions while
also preparing communities for the impacts of climate change. To address N2O,
USDA promotes efficient nitrogen stewardship to reduce over-application and
nitrogen runoff into waterways through the principles of right timing, right
fertilizer type, right placement, and right quantity. Adopting these techniques
will enable farmers to maintain yield while decreasing expenses on fertilizer.
Another Building Block supports livestock partnerships that use cost-share
support and technical assistance to encourage broader deployment of anaerobic
digesters, lagoon covers, composting, and solids separators to reduce methane
emissions from cattle, dairy, and swine operations.
Building on these actions, greater emissions reductions can be achieved through
broader uptake of these existing techniques, greater incentives to promote
climate-smart practices, and technological innovation. Investments in animal
genetics and breeding could improve the health and value of livestock while
reducing feed demand and decreasing livestock-related emissions. Safe food
additives like certain types of algae have the potential to significantly reduce
methane production in livestock (Kinley and Fredeen 2014). Small-scale anaerobic
digesters can capture methane from waste and supply renewable energy for
electricity and on-farm equipment. Slow-release fertilizers and other precision
agriculture techniques can reduce the amount of nitrogen that is applied to a
field. USDA estimates that we can reduce non-CO2 emissions from agriculture by
25 percent or more from current levels by 2050 by successfully expanding
existing mitigation options, making new technologies standard practice, and
expanding outreach and technical assistance efforts.22 Over time, many of these
mitigation solutions can lead to economic gains for farmers and ranchers,
including lower fertilizer costs, and increasing health and productivity of
livestock.
Addressing methane and N2O from agricultural production will continue to be
challenging. Risk aversion, highly competitive agricultural markets, and growing
impacts from climate change can make new practices unattractive for farmers and
ranchers. Achieving widespread adoption of these practices could require putting
in place economic incentives to help overcome potential concerns about lower
yields, lower profits, and any costs associated with new technologies and
practices. Improved approaches for capturing these emissions reductions in the
U.S. GHG Inventory are also needed. By no means are the opportunities laid out
in this report exhaustive. The MCS envisions additional focus on policies,
incentives, and innovative technologies to scale up non-CO2 mitigation from
agriculture.
METHANE AND NITROUS OXIDE FROM WASTE STREAMS
Landfills are the third largest source of
methane emissions in the United States, contributing 11 percent of non-CO2
emissions. As seen in Figure 6.2, landfill methane remains a significant share
of non-CO2 emissions in 2050 under the MCS Benchmark scenario. When organic
materials, such as food waste, decompose in the absence of oxygen, methane is
produced. Methane emissions are similarly generated from municipal and
industrial wastewater treatment activities, although centralized aerobic
wastewater treatment facilities limit the amount of methane released. Municipal
wastewater is also a source of N2O emissions—human
sewage emits N2O during both the nitrification and
denitrification of urea, ammonia, and proteins.
In July 2016, EPA finalized stringent standards to reduce methane emissions from
new and existing landfills that will result in
reductions of 8 million metric tons annually in 2025. The standard requires the
installation of gas
collection systems that capture methane to either flare or put to
productive use, such as powering on-site equipment. EPA also supports smaller
landfills in implementing
landfill gas capture through voluntary
programs like the Landfill Methane Outreach Program. The efficiency of biogas
collection systems is currently around 85 percent (EPA 2008). This may be
improved with technological advances and as new landfills
are designed with gas collection in mind. Some of the remaining fugitive
emissions from landfills could be reduced by
installing and maintaining bio-based systems such as bio covers or bio filters
that oxidize methane emissions.
While these measures can reduce methane once created, other actions can help
prevent methane production entirely. For
example, food waste reduction and diversion
programs cut the amount of organic waste decomposing in
landfills. Approximately 133 billion pounds of food
end up in landfills because it is either deemed
cosmetically unfit or will not stay fresh long enough to be shipped far
distances, making it the single greatest contributor to
municipal landfills (USDA 2015). In addition to
exacerbating methane emissions, food waste
contributes to excess fossil fuel and water use, while also putting increasing
pressures on cropland as global food demand grows. In September 2015, USDA and
EPA, along with many private sector and food bank partners, announced a national
target to reduce food waste 50 percent by 2030,
including through encouraging farmers to donate more of their imperfect produce
to the hungry (USDA 2015). Multiple states, including Massachusetts, Vermont,
and Connecticut, have implemented regulations to reduce food waste from
commercial sources (Perry 2014). Scaling up these waste diversion programs would
help significantly reduce landfill emissions in the future.
Finally, methane emissions in wastewater treatment
could be significantly reduced by 2050 through currently available mitigation
options, such as anaerobic biomass digesters and
centralized wastewater treatment facilities.
Improved operational practices, such as controlling dissolved oxygen levels
during treatment or limiting operating system upsets, can also help reduce N2O
emissions from wastewater treatment.
HFCs FROM REFRIGERATION AND AIR CONDITIONING
Fluorinated gases are man-made and used in a range of applications. They are
highly potent greenhouse gases, trapping hundreds to thousands of times more
heat than carbon dioxide. The vast majority of fluorinated gases emitted are
hydrofluorocarbons (HFCs). A substitute for ozone-depleting substances, HFCs are
primarily used for refrigeration and air conditioning.
Absent regulation, emissions of HFCs in the United States and globally were
expected to double between 2015 and 2030, due both to the phase-out of
ozone-depleting substances under the Montreal Protocol on Ozone-Depleting
Substances and the overall growth of air conditioning and refrigeration around
the world (EPA 2012, Velders et al. 2009). Fortunately, HFC emissions reductions
are achievable by preventing or reducing leaks and transitioning to the use of
low-GWP alternatives. The Obama Administration has reduced HFCs through both
international diplomacy and domestic actions.
Over the past several years, the Obama Administration announced a series of
executive actions to address HFCs. Under the Significant New Alternatives Policy
(SNAP), EPA lists acceptable alternatives used in aerosols, foam-blowing,
refrigeration, and other sectors. In 2015 and 2016, EPA finalized two
regulations to prohibit the use of certain HFCs and HFC-containing blends across
a variety of end-uses where safer and more climate-friendly alternatives are
available. In September 2016, EPA also finalized a regulation that would
strengthen existing refrigerant management requirements and extend safe
handling, reuse, and disposal requirements to HFCs.
Along with these regulatory measures, the White House announced a series of
private-sector commitments to cut HFC usage. The combination of private-sector
commitments and executive actions in the United States is estimated to reduce
domestic reliance on HFCs and contribute to a reduction in cumulative global
consumption by more than 1 billion MtCO2e through 2025.
Significant progress has also been made this year on the international front. In
October 2016, the United States worked with nearly 200 other countries to adopt
an amendment under the Montreal Protocol to phase down the production and
consumption of HFCs. Under the Kigali Amendment to the Montreal Protocol, the
United States and other countries listed under Article 2 of the Montreal
Protocol committed to phase down production and consumption of HFCs by 85
percent by 2036, while the rest of the world committed to 80 to 85 percent
reductions by 2047. The United States is also working with partners in the
Climate and Clean Air Coalition to Reduce Short-Lived Climate Pollutants (CCAC),
launched in 2012, to promote climate-friendly alternatives and standards for
HFCs.
Achieving HFC reductions beyond those shown in Figure 6.2 will depend on
addressing the existing stock of refrigerators and air conditioners, which
already contain HFCs and have potential to leak into the atmosphere over the
coming decades. For example, EPA can help to reduce or eliminate the leaking of
HFCs from various types of refrigerant-containing equipment through targeted
partnership programs such as its GreenChill program, which partners with food
retailers to, among other things, lower refrigerant charge sizes and eliminate
leaks. EPA can also scale up partnership programs such as the Responsible
Appliance Disposal Program to prevent emissions through the proper disposal of
appliances by ensuring recovery and reclamation or destruction of refrigerants
and foam.
Additional RD&D support to ensure new alternatives to HFCs continue to enter the
market may also be important, including both new molecules and new uses for
existing alternatives, though private sector players are already leading the way
on this front.
source
|